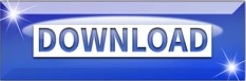
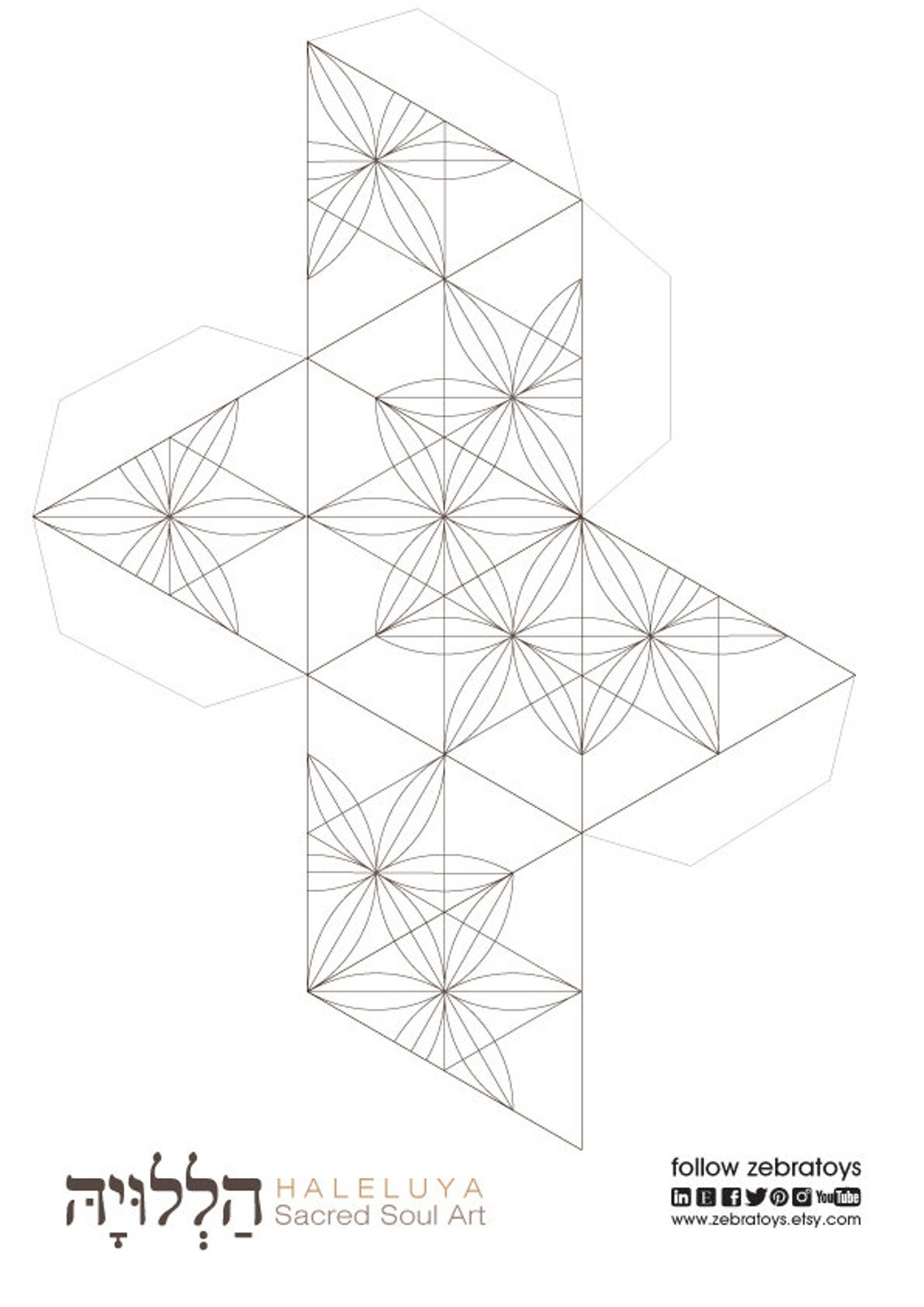
To demonstrate our ideas, we numerically and experimentally investigate the propagation of sound waves through the proposed reconfigurable metamaterial. Therefore, for (α 1, α 2, α 3) = (π/2, π/2, 0), we expect the system to behave as a classical single-tube waveguide, whereas for (α 1, α 2, α 3) = (π/3, 2π/3, π/3), we expect the additional wave interferences in the structure to result in a more complex frequency response and a variety of radiation patterns. 1, B and C), such that sound waves can only propagate in one direction. In this configuration, the tubes are acoustically coupled, and we expect the radiation by the structure to take place in all three directions, covering the entire surrounding space without a specific directivity. More specifically, for (α 1, α 2, α 3) = (π/2, π/2, π/2), the system is fully expanded and comprises a three-dimensional network of interconnected channels oriented in three perpendicular directions ( Fig. RESULTS Reconfigurable acoustic waveguides based on extruded cubes Here, we combine experiments and simulations to demonstrate that such three-dimensional networks of tubes can be exploited to design reconfigurable acoustic waveguides capable of efficiently controlling and redirecting the propagation of sound. These designs also result in interconnected and reconfigurable networks of tubes defined by the assembly of rigid plates and elastic hinges. Although most of the proposed origami-like metamaterials are based on two-dimensional folding patterns, snapology ( 15, 16)-a modular origami technique-has recently inspired the design of highly reconfigurable three-dimensional metamaterials assembled from extruded polyhedra ( 17). In particular, two-dimensional sheets folded along predefined creases have enabled the design of multistable structures ( 6– 9), materials with negative Poisson’s ratio ( 10– 12) and tunable stiffness ( 13), and topological metamaterials ( 14). Origami ( 5)-the ancient art of paper folding-not only results in intricate and aesthetically pleasant designs but also provides an ideal platform for the design of transformable mechanical metamaterials. Although these examples illustrate the potential of acoustic waveguides with more complex geometry, they only cover a small region of the available design space, and a natural question to ask is how the geometry of the three-dimensional networks of tubes affects the propagation of sound. Finally, three-dimensional networks of waveguides have been used to study sound propagation in regular urban areas ( 4). Moreover, it has also been shown that the propagation of acoustic waves in tubes arranged to form a square lattice can be successfully described with tools from solid-state physics and provides opportunities to control sound through dispersion and band gaps ( 3). Although most of the proposed acoustic waveguides consist of a single duct, it is well known that carefully connected tubes can result in significant transmitted noise reduction ( 1, 2). The proposed designs expand the ability of existing acoustic metamaterials and exploit complex waveguiding to enhance control over propagation and radiation of acoustic energy, opening avenues for the design of a new class of tunable acoustic functional systems.Īcoustic waveguides designed to direct sound are ubiquitous and can be found in cars, buildings, jet engines, medical devices, and musical instruments, to name just a few. Moreover, we demonstrate that the capability of the structure to guide and radiate acoustic energy along predefined directions can be easily switched on and off, as the networks of tubes are reversibly formed and disrupted. We design reconfigurable systems that, depending on the externally applied deformation, can act as networks of waveguides oriented along one, two, or three preferential directions. As such, they provide an ideal platform to actively control and redirect the propagation of sound. Our strategy builds on the fact that the rigid plates and hinges forming these structures define networks of tubes that can be easily reconfigured.
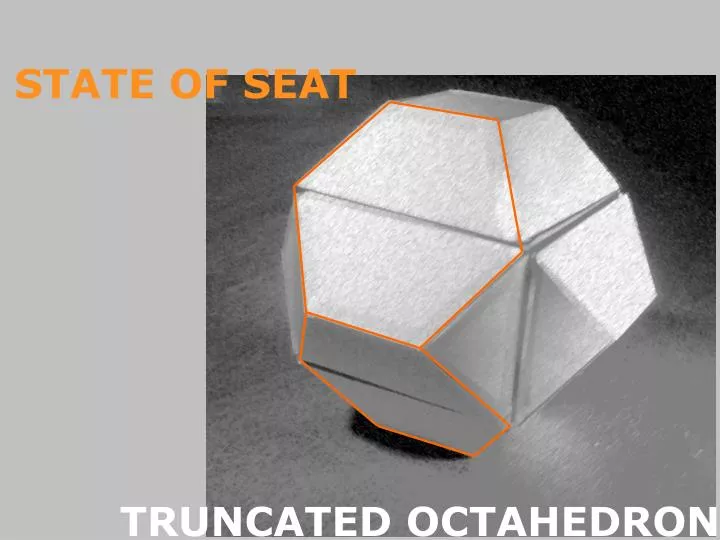
We combine numerical simulations and experiments to design a new class of reconfigurable waveguides based on three-dimensional origami-inspired metamaterials.
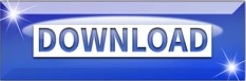